Volume 5 (2022) Special Edition No.1 Pages S1-S5
Abstract
Acute myeloid leukemia (AML), one of the most common hematological malignancies worldwide, is derived from a fraction of stem cells known as leukemic stem cells (LSCs), which possess self-renewal and high propagation capacities. Remaining quiescent and being resistant to conventional chemotherapy, residual LSCs after chemotherapy drive leukemia regrowth, leading to AML relapse. Therefore, the eradication of LSCs is critical for the treatment of AML. We previously identified hepatitis A virus cellular receptor 2 (HAVCR2/TIM-3) as an LSC-specific surface molecule by comparing gene expression in LSCs and hematopoietic stem cells (HSCs). TIM-3 expression clearly discriminated LSCs from HSCs within the CD34+CD38− stem cell fraction. Furthermore, AML cells secrete galectin-9, a TIM-3 ligand, in an autocrine manner, leading to constitutive TIM-3 signaling that maintains the self-renewal capacity of LSCs via the induction of β-catenin accumulation. Thus, TIM-3 is an indispensable functional molecule for human LSCs. Herein, we review the functional aspects of TIM-3 in AML and evaluate minimal/measurable residual disease with a focus on CD34+CD38−TIM-3+ LSCs. Using sequential genomic analysis of identical patients, we determined that CD34+CD38−TIM-3+ cells in the complete remission (CR) phase after allogeneic stem cell transplantation (allo-SCT) are the LSCs responsible for AML relapse. We retrospectively evaluated the incidence of TIM-3+ residual LSCs. All analyzed patients achieved CR and complete donor chimerism at the engraftment phase; however, the high frequency of residual TIM-3+ LSCs within the CD34+CD38− fraction at engraftment was a significant and independent risk factor for relapse. Residual TIM-3+ LSC levels in the engraftment phase had a stronger impact on relapse than did pre-SCT disease status. Therefore, the evaluation of residual TIM-3+ LSCs is a promising approach for predicting leukemia relapse after allo-SCT.
1. Introduction
Acute myeloid leukemia (AML) originates from a small fraction of self-renewing leukemic stem cells (LSCs), which can repopulate human AML in immunodeficient mice after xenogeneic transplantation1,2. Leukemic stem cells possess self-renewal capacity and differentiation potential toward leukemic blasts, thereby maintaining the AML population. Acute myeloid leukemia LSCs were originally identified in the CD34+CD38− fraction, and their phenotype is identical to that of normal hematopoietic stem cells (HSCs). Recent research advances in immunodeficient mice have enabled the isolation of LSCs from the CD34+CD38− fraction3; however, quiescent LSCs with high self-renewal potential are concentrated within this fraction4. Given that LSCs originate from multipotent self-renewing pre-leukemic HSCs, in which somatic mutations or genetic events accumulate throughout their life cycle5-7, LSCs and HSCs share multiple molecular mechanisms for the maintenance of their stemness. Such similarities between LSCs and HSCs have hampered the development of LSC-specific therapeutic strategies while sparing the HSCs. We intensively investigated the gene expression of FACS-purified CD34+CD38− HSCs and LSCs and identified T-cell immunoglobulin mucin-3 (TIM-3) as an LSC-specific surface molecule whose expression was not observed in HSCs. TIM-3 expression clearly discriminates LSCs from HSCs within the CD34+CD38− stem cell fraction8.
In this review, we discuss the function of TIM-3 in AML LSCs. Furthermore, we discuss the significance of the evaluation of minimal/measurable residual disease (MRD), focusing on CD34+CD38−TIM-3+ LSCs in allogeneic stem cell transplantation (allo-SCT).
2. Role of TIM-3 in Hematopoiesis
TIM-3 belongs to the TIM family, and HAVCR1, HAVCR2, and TIMD4 encode TIM-1, TIM-3, and TIM-4, respectively. TIM-3 consists of an N-terminal immunoglobulin (Ig) variable domain, mucin domain, transmembrane domain, and cytoplasmic tail. The cytoplasmic tail of TIM-3 contains conserved tyrosine residues and an Src homology 2 (SH2)-binding motif.
TIM-3 was originally identified as a 60 kDa surface molecule expressed on interferon-γ-producing CD4+ and CD8+ T cells in mice. Previous studies have demonstrated that TIM-3 is expressed in other types of blood cells such as monocytes, dendritic cells (DCs),
Four independent ligands of TIM-3 have been identified: galectin-9 (gal-9), phosphatidylserine (PtdSer), high-mobility group box 1 (HMGB1), and carcinoembryonic antigen-related cell adhesion molecule 1 (CEACAM1)9. Gal-9, an S-type lectin, is expressed and secreted by hematopoietic cells. Gal-9 ligation to TIM-3 induces apoptosis of Th1 cells and inhibits interferon-γ production11. PtdSer has been identified as a common ligand for all TIM family members based on the crystal structure analysis of TIM-1, TIM-3, and TIM-412, 13. TIM-3 plays crucial roles in the phagocytosis of apoptotic cells and cross-presentation by DCs via its interaction with PtdSer14. CEACAM1 is considered to bind to the cleft framed by the FG and CC loops of the IgV domain of TIM-3, similar to PtdSer15. HMGB1 was identified as a TIM-3 ligand in DCs; TIM-3+ tumor-infiltrating DCs attenuated the nucleic acid-mediated innate immune response by interacting with TIM-3 and HMGB116.
The interaction between ligands and TIM-3 results in the recruitment of Src family kinases to the SH2 binding motif of the cytoplasmic tail, leading to downstream signal transduction. In general, ligation of TIM-3 ligands suppresses the activation of TIM-3+ T cells; therefore, TIM-3 has been extensively investigated as a
Consistent with the suppressive function of TIM-3 in T cells, the most dysfunctional T-cell subset of tumor-infiltrating CD8+PD-1+ T cells expresses TIM-317–19. The double blockade of programmed cell death 1 and TIM-3 by monoclonal antibodies showed a synergistic effect against tumors in murine models17 and improved the tumor antigen-specific human CD8+ T-cell response in vitro18, 19. Furthermore, recent studies have emphasized the significance of TIM-3 in tumor immunity by modifying Treg function, with the majority of tumor-infiltrating human Treg cells expressing TIM-320, 21. Accordingly, antibody blockade of TIM-3 suppresses Treg function22 and attenuates tumor growth23 in mice. Based on the results of these studies, anti-human TIM-3 monoclonal antibodies have been developed as
3. TIM-3 Expression Marks Functional LSCs in Human Myeloid Malignancies
TIM-3 has been extensively investigated as a negative regulator of T-cell function. However, unlike other co-inhibitory molecules, TIM-3 lacks known inhibitory signaling motifs in the cytoplasmic domain. Therefore, we attempted to identify the LSC-specific functions of TIM-3 in AML cells. We first evaluated the serum concentration of gal-9, the most extensively investigated ligand of TIM-3, in humans and unexpectedly found that patients with AML exhibited a significant increase in serum gal-9 levels compared to healthy donors and patients with other hematological malignancies, such as acute lymphoblastic leukemia and lymphoma. Based on these observations, we hypothesized that TIM-3+ AML cells secrete gal-9 in an autocrine manner in humans. Consistently, xenotransplantation of primary TIM-3+ LSCs reconstituted human AML in recipient mice with high serum concentrations of human gal-9. Blockade of the TIM-3/gal-9 interaction using an anti-human gal-9 monoclonal antibody strongly suppressed the leukemia reconstitution potential of AML LSCs in xenotransplantation experiments, suggesting a critical role of the TIM-3/gal-9 autocrine loop in the maintenance of LSC properties25. Furthermore, we found that TIM-3/gal-9 interaction promoted the co-activation of the nuclear factor (NF)-κB and β-catenin pathways in human AML cells. Nuclear accumulation of β-catenin and subsequent activation of its target genes are involved in the maintenance of LSCs in murine AML models26 and human chronic myelogenous leukemia27. Furthermore, co-activation of NF-κB and β-catenin coordinately promotes the development of cancer stem cells in a murine colorectal cancer model28. Therefore, we hypothesized that this TIM-3/gal-9 autocrine loop may be involved in the development of human myeloid LSCs. In humans, the frequencies of TIM-3+ aberrant HSCs within the CD34+CD38- fraction increased along with disease progression toward AML in myeloid malignancies, including myelodysplastic syndrome and myeloproliferative neoplasm25. Importantly, purified-CD34+CD38−TIM-3+ cells exclusively reconstituted AML, whereas CD34+CD38−TIM-3− cells from identical patients reconstituted multi-lineage human normal hematopoiesis in xenotransplantation experiments29, suggesting that TIM-3 clearly discriminates functional LSCs from HSCs. Consistent with the distinct reconstitution potential of CD34+CD38−TIM-3+ LSCs and CD34+CD38−TIM-3− HSCs, genetic analysis revealed a clonal relationship between CD34+CD38−TIM-3+ and CD34+CD38−TIM-3− cells; CD34+CD38−TIM-3+ LSCs represented clones harboring driver mutations derived from CD34+CD38−TIM-3− cells containing pre-leukemic HSCs6. Collectively, these results suggest that TIM-3 is a functional molecule required to maintain the properties of human myeloid LSCs.
We identified various unique molecules and downstream signaling cascades involved in TIM-3 signaling in AML LSCs (Sakoda, Kikushige et al., under revision). Interestingly, the downstream molecules in TIM-
4. Therapeutic Strategies Targeting TIM-3 in Human Myeloid Malignancies
We previously reported that a cytotoxic anti-human TIM-3 monoclonal antibody effectively killed LSCs without affecting normal human hematopoiesis in vivo8.
A recent study demonstrated the efficacy of bispecific chimeric antigen receptor T cells targeting both CD13 and TIM-3 in the treatment of human AML in vivo30.
In addition to cytotoxic therapeutic approaches against TIM-3+ AML cells, Recent clinical studies have demonstrated the efficacy of blocking TIM-3+ myeloid malignancies. As anti-human TIM-3 monoclonal antibodies have been developed as immune checkpoint inhibitors, first-in-human phase I/II studies have been initiated to test the safety and efficacy of these antibodies in cancer treatment24. The efficacy of anti-human TIM-3 monoclonal antibodies has been evaluated in combination with other immune checkpoint inhibitors such as anti-PD-1/PD-L1 monoclonal antibodies31. Sabatolimab (MBG453), an anti-human TIM-3 humanized IgG4 monoclonal antibody with the S228P mutation, has been tested for the treatment of myeloid malignancies, including AML, high-risk myelodysplastic syndromes, and chronic myelomonocytic leukemia. Combination therapy with sabatolimab and hypomethylating agents (HMAs) has shown preliminary efficacy and safety in recent clinical trials32.
5. TIM-3 Marks Residual Leukemic Stem Cells Responsible for Relapse in allo-SCT
Acute myeloid leukemia relapse remains a major clinical challenge. Particularly, the prognosis of patients who experience relapse after allo-SCT is poor. Leukemic stem cells remain quiescent and are resistant to conventional chemotherapy. Residual LSCs after chemotherapy eventually drive leukemia regrowth, leading to AML relapse. Therefore, the evaluation of MRD, focusing on LSCs, can be a powerful strategy to improve the clinical outcome of AML. Consistently, the European Leukemia Network MRD Working Party recommends the evaluation of LSC markers in multiparameter flow cytometry-based MRD in addition to the conventional leukemia-associated immunophenotype and different-from-normal-based staining strategies33. TIM-3 clearly discriminated LSCs from HSCs within the CD34+CD38− stem cell fraction. In addition, TIM-3 expression in AML cells is stably preserved at the time of relapse34, 35. Based on these data, we consider TIM-3 an ideal surface marker for LSCs in MRD evaluation.
We conducted a retrospective cohort study to investigate whether the evaluation of residual LSCs using TIM-3 expression could predict AML relapse after allo-SCT. The frequency of residual TIM-3+ LSCs within the CD34+CD38− fraction at the time of engraftment was strongly correlated with relapse after allo-SCT, although all patients achieved hematological complete remission (hCR) and complete donor chimerism. The levels of residual TIM-3+ LSCs in the engraftment phase had a stronger impact on relapse than did pre-SCT disease status, indicating the significance of response-oriented MRD evaluation strategies. Furthermore,
6. Conclusion
TIM-3 expression clearly discriminated donor-derived normal HSCs and recipient-derived LSCs within the CD34+CD38− cellular fraction. Therefore, evaluation of CD34+CD38−TIM-3+ residual LSCs after allo-SCT would be a useful method for the risk stratification of AML relapse, as discussed in this review. Furthermore, the blockade of TIM-3 signaling represents a unique therapeutic strategy to eradicate LSCs via two independent mechanisms: blockade of the TIM-3/gal-9 autocrine loop in TIM-3+ AML and restoration of T cell immunity against AML. Thus, the combination of TIM-3-targeted therapeutic approaches and TIM-3-based MRD assessment may be used to improve the clinical outcomes of patients with AML after allo-SCT. Further studies are necessary to determine the clinical role of TIM-3 in myeloid malignancies.
Funding Statement
This study was supported in part by a Grant-in-Aid for Scientific Research (B) (to Y.K. No. 22494899) and a Grant-in-Aid for challenging Exploratory Research (to Y.K. No.20269344) from Ministry of Education, Culture, Sports, Science and Technology of Japan.
The author has the following financial relationships to disclose: Patents royalties/gain from Novartis International AG.
Conflicts of Interest
The author declares no conflict of interest. a disclosure form provided by the author is available on the website.
References
1.Lapidot T, Sirard C, Vormoor J, Murdoch B, Hoang T, Caceres-Cortes J, et al. A cell initiating human acute myeloid leukaemia after transplantation into SCID mice. Nature. 1994; 367: 645-8.
2.Bonnet D, Dick JE. Human acute myeloid leukemia is organized as a hierarchy that originates from a primitive hematopoietic cell. Nat Med. 1997; 3: 730-7.
3.Goyama S, Wunderlich M, Mulloy JC. Xenograft models for normal and malignant stem cells. Blood. 2015; 125: 2630-40.
4.Ishikawa F, Yoshida S, Saito Y, Hijikata A, Kitamura H, Tanaka S, et al. Chemotherapy-resistant human AML stem cells home to and engraft within the bone-marrow endosteal region. Nat Biotechnol. 2007; 25: 1315-21.
5.Miyamoto T, Weissman IL, Akashi K. AML1/ETO-expressing nonleukemic stem cells in acute myelogenous leukemia with 8;21 chromosomal translocation. Proc Natl Acad Sci U S A. 2000; 97: 7521-6.
6.Jan M, Snyder TM, Corces-Zimmerman MR, Vyas P, Weissman IL, Quake SR, et al. Clonal evolution of preleukemic hematopoietic stem cells precedes human acute myeloid leukemia. Sci Transl Med. 2012; 4: 149ra118.
7.Shlush LI, Zandi S, Mitchell A, Chen WC, Brandwein JM, Gupta V, et al. Identification of pre-leukaemic haematopoietic stem cells in acute leukaemia. Nature. 2014; 506: 328-33.
8.Kikushige Y, Shima T, Takayanagi S, Urata S, Miyamoto T, Iwasaki H, et al. TIM-3 is a promising target to selectively kill acute myeloid leukemia stem cells. Cell Stem Cell. 2010; 7: 708-17.
9.Kikushige Y. TIM-3 in normal and malignant hematopoiesis: Structure, function, and signaling pathways. Cancer Sci. 2021; 112: 3419-26.
10.Kikushige Y, Miyamoto T. Identification of TIM-3 as a Leukemic Stem Cell Surface Molecule in Primary Acute Myeloid Leukemia. Oncology. 2015; 89 Suppl 1: 28-32.
11.Zhu C, Anderson AC, Schubart A, Xiong H, Imitola J, Khoury SJ, et al. The Tim-3 ligand galectin-9 negatively regulates T helper type 1 immunity. Nat Immunol. 2005; 6: 1245-52.
12.Kobayashi N, Karisola P, Pena-Cruz V, Dorfman DM, Jinushi M, Umetsu SE, et al. TIM-1 and TIM-4 glycoproteins bind phosphatidylserine and mediate uptake of apoptotic cells. Immunity. 2007; 27: 927-40.
13.DeKruyff RH, Bu X, Ballesteros A, Santiago C, Chim YL, Lee HH, et al. T cell/transmembrane, Ig, and mucin-3 allelic variants differentially recognize phosphatidylserine and mediate phagocytosis of apoptotic cells. J Immunol. 2010; 184: 1918-30.
14.Nakayama M, Akiba H, Takeda K, Kojima Y, Hashiguchi M, Azuma M, et al. Tim-3 mediates phagocytosis of apoptotic cells and cross-presentation. Blood. 2009; 113: 3821-30.
15.Huang YH, Zhu C, Kondo Y, Anderson AC, Gandhi A, Russell A, et al. CEACAM1 regulates TIM-3-mediated tolerance and exhaustion. Nature. 2015; 517: 386-90.
16.Chiba S, Baghdadi M, Akiba H, Yoshiyama H, Kinoshita I, Dosaka-Akita H, et al. Tumor-infiltrating DCs suppress nucleic acid-mediated innate immune responses through interactions between the receptor TIM-3 and the alarmin HMGB1. Nat Immunol. 2012; 13: 832-42.
17.Sakuishi K, Apetoh L, Sullivan JM, Blazar BR, Kuchroo VK, Anderson AC. Targeting Tim-3 and PD-1 pathways to reverse T cell exhaustion and restore anti-tumor immunity. J Exp Med. 2010; 207: 2187-94.
18.Fourcade J, Sun Z, Pagliano O, Chauvin JM, Sander C, Janjic B, et al. PD-1 and Tim-3 regulate the expansion of tumor antigen-specific CD8(+) T cells induced by melanoma vaccines. Cancer Res. 2014; 74: 1045-55.
19.Fourcade J, Sun Z, Benallaoua M, Guillaume P, Luescher IF, Sander C, et al. Upregulation of Tim-3 and PD-1 expression is associated with tumor antigen-specific CD8+ T cell dysfunction in melanoma patients. J Exp Med. 2010; 207: 2175-86.
20.Gao X, Zhu Y, Li G, Huang H, Zhang G, Wang F, et al. TIM-3 expression characterizes regulatory T cells in tumor tissues and is associated with lung cancer progression. PLoS One. 2012; 7: e30676.
21.Yan J, Zhang Y, Zhang JP, Liang J, Li L, Zheng L. Tim-3 expression defines regulatory T cells in human tumors. PLoS One. 2013; 8: e58006.
22.Sakuishi K, Ngiow SF, Sullivan JM, Teng MW, Kuchroo VK, Smyth MJ, et al. TIM3(+)FOXP3(+) regulatory T cells are tissue-specific promoters of T-cell dysfunction in cancer. Oncoimmunology. 2013; 2: e23849.
23.Liu JF, Wu L, Yang LL, Deng WW, Mao L, Wu H, et al. Blockade of TIM3 relieves immunosuppression through reducing regulatory T cells in head and neck cancer. J Exp Clin Cancer Res. 2018; 37: 44.
24.Qin S, Xu L, Yi M, Yu S, Wu K, Luo S. Novel immune checkpoint targets: moving beyond PD-1 and CTLA-4. Mol Cancer. 2019; 18: 155.
25.Kikushige Y, Miyamoto T, Yuda J, Jabbarzadeh-Tabrizi S, Shima T, Takayanagi S, et al. A TIM-3/Gal-9 Autocrine Stimulatory Loop Drives Self-Renewal of Human Myeloid Leukemia Stem Cells and Leukemic Progression. Cell Stem Cell. 2015; 17: 341-52.
26.Wang Y, Krivtsov AV, Sinha AU, North TE, Goessling W, Feng Z, et al. The Wnt/beta-catenin pathway is required for the development of leukemia stem cells in AML. Science. 2010; 327: 1650-3.
27.Jamieson CH, Ailles LE, Dylla SJ, Muijtjens M, Jones C, Zehnder JL, et al. Granulocyte-macrophage progenitors as candidate leukemic stem cells in blast-crisis CML. N Engl J Med. 2004; 351: 657-67.
28.Schwitalla S, Fingerle AA, Cammareri P, Nebelsiek T, Goktuna SI, Ziegler PK, et al. Intestinal tumorigenesis initiated by dedifferentiation and acquisition of stem-cell-like properties. Cell. 2013; 152: 25-38.
29.Jan M, Chao MP, Cha AC, Alizadeh AA, Gentles AJ, Weissman IL, et al. Prospective separation of normal and leukemic stem cells based on differential expression of TIM3, a human acute myeloid leukemia stem cell marker. Proc Natl Acad Sci U S A. 2011; 108: 5009-14.
30.He X, Feng Z, Ma J, Ling S, Cao Y, Gurung B, et al. Bispecific and split CAR T cells targeting CD13 and TIM3 eradicate acute myeloid leukemia. Blood. 2020; 135: 713-23.
31.Wolf Y, Anderson AC, Kuchroo VK. TIM3 comes of age as an inhibitory receptor. Nat Rev Immunol. 2020; 20: 173-85.
32.Zeidan AM, Komrokji RS, Brunner AM. TIM-3 pathway dysregulation and targeting in cancer. Expert Rev Anticancer Ther. 2021; 21: 523-34.
33.Schuurhuis GJ, Heuser M, Freeman S, Béné MC, Buccisano F, Cloos J, et al. Minimal/measurable residual disease in AML- a consensus document from the European LeukemiaNet MRD Working Party. Blood. 2018; 131: 1275-91.
34.Haubner S, Perna F, Köhnke T, Schmidt C, Berman S, Augsberger C, et al. Coexpression profile of leukemic stem cell markers for combinatorial targeted therapy in AML. Leukemia. 2019; 33: 64-74.
35.Ho TC, LaMere M, Stevens BM, Ashton JM, Myers JR, O'Dwyer KM, et al. Evolution of acute myelogenous leukemia stem cell properties after treatment and progression. Blood. 2016; 128: 1671-8.
Search
News
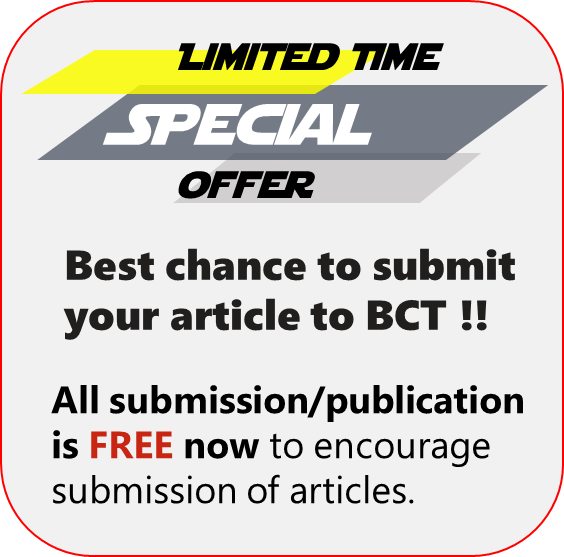